Electron Beam Melting (EBM) is an additive manufacturing process that uses an electron beam to selectively melt metal powder layer-by-layer to build up fully dense parts. ebm manufacturing process offers capabilities beyond conventional manufacturing methods for producing complex, high-performance metal parts.
Overview of ebm manufacturing process
EBM works similarly to other powder bed fusion techniques. The process takes place in a high vacuum chamber where an electron beam selectively scans and melts powder spread in thin layers on a build plate. After each layer is melted, the build plate lowers and more powder is raked over the surface, then the electron beam melts and fuses the next layer.
Key Details:
- Build Parts Layer-by-Layer from Metal Powder
- Electron Beam Selectively Melts Powder
- Process Takes Place in a Vacuum
- High Beam Power for Rapid Melting
- Support Structures Used, Removed Post-Process
- Repeats Until Complete Part Formed
Benefits:
- Design Freedom for Complex Geometries
- Functional Metal Parts Direct from CAD
- Excellent Mechanical Properties
- High Density Parts, Up to 99.9%
- Reduce Waste Compared to Machining
EBM provides greater freedom to manufacture complex geometries with fewer restrictions on angles, overhangs and undercuts compared to traditional fabrication. Parts produced via EBM offer mechanical properties comparable or superior to wrought properties.
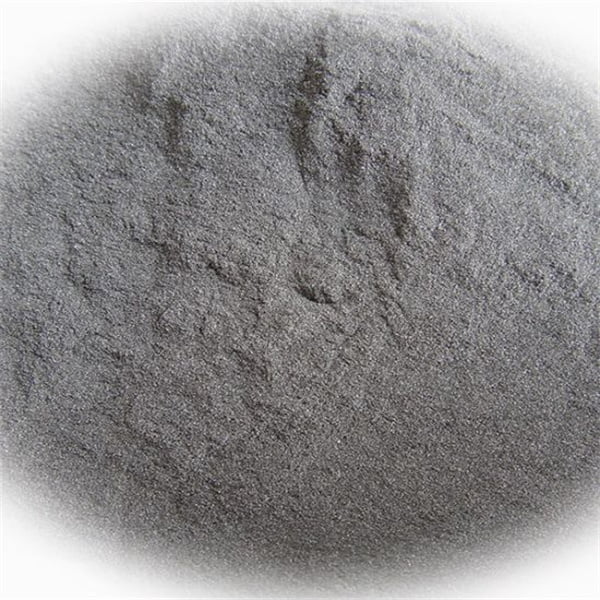
Materials Used in EBM
EBM is capable of processing various alloys into fully dense parts, with most usage focused on titanium, aluminum, cobalt chrome, nickel alloys, stainless steels, and tool steels.
Materials:
- Titanium Ti64, Ti64ELI, commercially pure titanium
- Aluminum AlSi10Mg, AlSi12, Scalmalloy
- Cobalt Chrome CoCrMo, CoCrW
- Nickel Alloys IN718, IN625, IN939
- Stainless Steels 316L, 17-4PH, 304L, 420
- Tool Steels H13, D2, M2
- Others: CuSn10, CuCr1Zr
Titanium alloys see heavy use in aerospace components along with nickel superalloys. Cobalt chrome is popular for medical implants. Tool steel molds and aluminum components serve automation and automotive. Various metal powders down to 15 microns in size can be utilized.
EBM Process Capabilities
EBM can directly manufacture end-use parts and products ready for service with little to no post-processing. Some key capabilities include:
Geometric Complexity
- Complex lattices and mesh structures
- Deep channels, undercuts, tunnels
- Thin walls (< 1 mm) and fine details
- Lightweighting topology optimization
Mechanical Properties
- Strength and hardness values match or exceed wrought metals
- Fatigue and fracture resistance on par with wrought
- Dense components with porosity under 0.8%
Accuracy & Resolution
- Accuracy to within 0.2 mm or 2% of part dimension
- Finest feature size around 0.3 mm
- Thinnest walls of 0.25-0.5 mm
- Minimum feature sizes continue improving
Surface Finish
- Raw EBM surface finish around 5-9 μm roughness
- Finish profiling can achieve 1.5 μm roughness
- Additional processes used for finer polishing
Build Volumes
- Commercial systems range 150 mm diameter up to 1000 x 600 x 500 mm builds
- Larger customs systems also in development
- Continual improvements in maximum part sizes
Steps of the EBM Additive Manufacturing Process
The EBM manufacturing process takes place as a series of steps to setup, prepare, build, and complete metal parts additively layer-by-layer using an electron beam to melt and fuse material.
EBM Process Steps:
- 3D Model Part and Optimize Design for AM
- Convert File to Standard Format for EBM System
- Select Material, Set Build Parameters
- Prepare Metal Powder according to Specifications
- Load Powder in Machine, Level Build Area
- Spread Powder Layer, Pre-heat with Beam
- Hatch Melt Areas for Each Layer, Fuse Metal
- Lower Platform, Add Next Layer
- Repeat Layering/Melting to Complete Part
- Remove from Chamber, Separate Part from Base Plate
- Remove Support Structures from Part
- Post-Process via Machining, Polishing, Hot Isostatic Pressing as Needed
Critical process parameters optimized for each material include beam power, beam speed, hatch spacing, scan strategy, layer time, layer thickness, preheat temps, and post-process heat treatments. Tuning these settings allows adjusting part density, residual stress, surface finish, microstructure, and mechanical properties.
Advantages of Electron Beam Additive Manufacturing
EBM provides several compelling advantages over traditional subtractive fabrication or other additive methods in terms of cost, performance, efficiency, complexity, properties, and sustainability.
Advantages of EBM:
- Design freedom for lightweight, complex geometries
- Streamlined workflow from CAD to finished functional part
- Eliminates tooling, fixturing for complex features
- High metal density up to 99.9%
- Excellent strength and hardness properties
- Lower cost per part for small/medium volumes
- Reduced waste metal compared to subtractive processes
- Minimizes post-processing and lead times
- Parameter control for microstructure and properties
- High beam power enables faster build speeds
The EBM process facilitates innovative design approaches and consolidation of complex parts for improved performance. It rapidly produces highly dense end-use metal components without excessive cost associated with specialty tooling or extensive finishing operations.
Limitations of Electron Beam Additive Manufacturing
Alongside the advantages, EBM has some inherent limitations to consider as well when determining suitability for production applications.
Limitations of EBM:
- Higher equipment cost than polymer systems
- Limited approved aerospace/medical alloys currently
- Beam deflection optics limit maximum build size
- Lower scanning speeds than laser powder bed fusion
- Lower fine-feature resolution than laser systems
- Beam melting affected by powder conductivity and build height
- Post-processing to improve surface finish
- Small internal voids or lack of fusion defects possible
- Process monitoring and quality control areas to mature
The elevated equipment expenses associated with electron beam systems results in higher part costs, making EBM more suitable for lower volume production where cost savings from tooling offsets initial capital outlays.
Quality Control and Post-Processing in EBM
As with all powder bed metal additive manufacturing processes, EBM components can face potential quality issues regarding porosity, anisotropic properties, surface finish, residual stress, powder effects, and geometric dimensioning that require mitigation through parameter optimization, post-processing, hot isostatic pressing, and quality control procedures.
Quality Control:
- Consistent feedstock metal powder properties
- Parameter optimization specific to alloy and geometry
- In situ monitoring of thermal emissions
- Post-build CT scans to verify densities
- Testing of mechanical properties per ASTM standards
Post-Processing:
- Thermal stress relief and hot isostatic pressing
- Bead blasting to improve surface finish
- Milling, turning, grinding, polishing
- Coatings or treatments for functional properties
Ongoing efforts continue advancing real-time process monitoring, feedstock powder quality control, modeling simulations, and closed-loop feedback parameter optimization to progress quality and reliability.
Applications of Electron Beam Additive Manufacturing
The design freedoms unlocked by EBM translate to high-performance end-use components across progressive industries pushing boundaries in aerospace, defense, medical technology, automotive, and energy applications.
Industry Applications:
Aerospace – Turbine blades, impellers, thrust chambers, lattices Automotive – Lightweighting chassis and powertrain components Medical – Orthopedic implants, prosthetics devices
Energy – Heat exchangers, pressure vessels, drilling Defense – UAVs, protective military vehicle parts Tooling – Conformal cooling molds, dies, patterns
EBM facilitates consolidation of complex assemblies into single parts with enhanced properties and serves small to medium batch production. The process continues progressing from rapid prototyping towards certified serial fabrication as broader alloys and quality control measures mature.
Suppliers of EBM Equipment
A handful of established industrial providers offer commercial EBM additive manufacturing systems along with integrated powder handling solutions. Custom large-format machines also under development.
EBM Equipment Suppliers:
Supplier | Model | Build Size | Materials |
---|---|---|---|
Arcam EBM | Arcam Q20plus | Ø350 x 380 mm | Ti alloys, CoCr, Al, steels, Ni alloys |
Arcam EBM Spectra H | 650 x 400 x 500 mm | Tool steel, stainless steel, CoCrMo, AlSi10Mg | |
Arcam EBM Spectra L | 350 x 350 x 380 mm | Ti alloys, Co alloys, Ni alloys, steels | |
JEOL | JAM-1000 | 300 x 300 x 300 mm | Ti alloys, Ni alloys, Cu alloys, steels |
Sciaky | EBAM 300 | 3050 x 1220 x 1220 mm | Al alloys, aerospace certified |
Wayland Additive | Calibur3 | Ø685 x 380 mm | Ti64, Inconel 718, stainless steels |
Small Scale / Research Systems
- Arcam A2X
- Institute of Advanced Manufacturing Technology EBAM 150
- Linz Center of Mechatronics GmbH Micro-EBAM
Larger build volumes continue to increase with customized solutions above one meter lengths under R&D. Beam widths also progressing beyond single e-beam towards multiple coordinated beams for faster speeds.
Cost Analysis of Electron Beam Additive Manufacturing
Adopting EBM as an end-use metal additive manufacturing process requires weighing expenses for equipment acquisition, material consumables, and operational overheads against unit part cost savings from consolidating assemblies, minimizing machining, and right-sizing inventories.
Cost Factors – EBM vs Traditional Manufacturing
Cost Factor | EBM | Subtractive Manufacturing |
---|---|---|
Equipment Acquisition | $500K – $1.5M+ | Lower machinery expenses |
Material Powders | $100 – $800 per kg | Lower raw material costs |
Labor | $50 – $150 per hour | Lower labor rates typically |
Build Rate | 5-20 cm3/hour for production | Higher volumes per hour |
Energy Consumption | ~50 kWh per kg built | Lower energy usage |
Supply Chain | Simplify logistics, reduce lead times | Complex global supply chains |
Per Part Cost | $2 – $500+, depends on size/geometry/quantity | Highly geometry dependent |
Total Cost | $5K – $25K+ for build volume per year | Highly variable per industry |
In general, EBM provides advantages for lower volume complex metal parts around one to a few hundred units where inventory, machining time, and assembly consolidations offset order-of-magnitude higher machine and powder costs relative to high-volume traditional manufacturing with expensive tooling and extreme batch sizes. Part quantity, rate, complexity, performance targets, and quality expectations factor heavily into optimal production method selection.
Safety Considerations for Electron Beam Additive Manufacturing
As with all industrial manufacturing equipment, electron beam metal 3D printing introduces health and safety risks regarding high voltage electricity, inert gases, reactive metal powders, and handling of toxic materials requiring hazard mitigation controls.
EBM Safety Considerations:
- High voltage electronics containment
- Shielding from x-ray exposures
- Cryogenic gases under pressure
- Reactive metal powder dusts
- Nanoparticle exposures
- Manual powder sieving ergonomics
- Mechanical and laser interlocks
- Material flammability precautions
- Staff PPE requirements
- Equipment lockouts and e-stops
- Routine maintenance for uptime
- Monitoring of beam hours and performance
Thorough operator training combined with engineering controls, safety protocols, protective equipment, regulated maintenance, and Assembly Bill & Conformité Européenne directives enforced for powder bed equipment provide multiple layers of health and environmental protections.

Future Outlook for Electron Beam Additive Manufacturing
As EBM technology and quality continue maturing, broader adoption is expected across aerospace, medical, industrial, tooling, and automotive sectors favoring applications with moderate production volumes under a few thousand units where performance justifies higher costs.
Future Trends in EBM:
- Expanding portfolio of processable alloys
- Hardware for larger build volumes
- Multi-beam systems for faster speeds
- Enhanced beam deflection range and accuracy
- Improved beam focusing and precision
- In situ monitoring and closed-loop control
- Standardization of process parameters
- Comprehensive powder management solutions
- Hybrid manufacturing integration
- Quality benchmarks for certification
- High-rate production applications
Incorporating multiple coordinated electron beams can multiply scanning speeds. Hybrid systems combining EBM with milling or other secondary operations in a single platform enable streamlined post-machining. As hardware, materials, quality protocols, and part approval workflows progress, EBM adoption suits higher rate production across expanding certified applications.
FAQ
Q: What materials can EBM process?
A: EBM commonly processes titanium, aluminum, nickel, cobalt chrome, stainless steel, tool steel, and copper alloys. Material options continue expanding.
Q: Does EBM produce porous or fully dense parts?
A: EBM produces over 99% dense metal components using partially pre-sintered powders. Density exceeds cast and wrought metals.
Q: How accurate is EBM compared to other metal AM processes?
A: Dimensional accuracy reaches ±0.2 mm with tolerances competitive with other powder bed technologies, precision improves with experience.
Q: What industries use EBM manufacturing?
A: Aerospace, medical, automotive, industrial, tooling, defense, robotics, energy industries leverage EBM for end-use components.
Q: What is the cost per part for EBM additive manufacturing?
A: Part costs vary from $100 to $10,000+ depending on size, geometry complexity, build rates, material volumes, post-processing needs, etc.
Q: What service providers offer EBM additive manufacturing?
A: RapidDirect, 3D Systems, Carpenter Additive, Alloyed, Sigma Labs, Velo3D, Barnes Aerospace, Burloak Technologies, Morf3D.